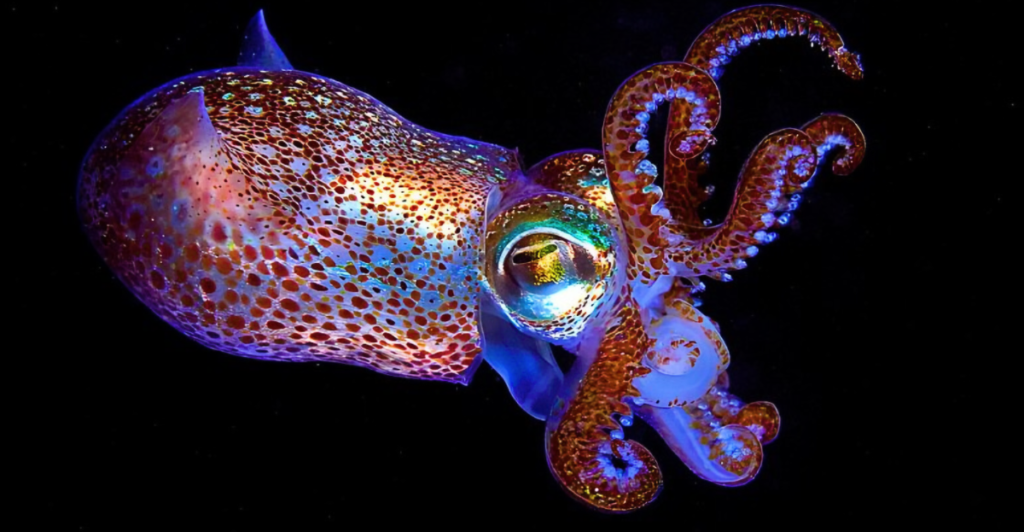
Beneath the waves, marine life is rewriting what we know about light and energy. Cephalopods like squids and octopuses have evolved the ability to change their skin color in milliseconds, thanks to sophisticated pigment systems called chromatophores. But this biological feat is more than camouflage—it’s a lesson in efficient light manipulation.
Researchers at Northeastern University have discovered that these pigment granules aren’t just visual—they can also convert light into electricity, even under low-light aquatic conditions. With photoconversion efficiencies reaching 0.81±0.14%, squid-derived materials may hold the key to solar panels that function where traditional ones fail.
This comes at a pivotal moment, as the maritime sector aims to slash emissions by 50% by 2050 under International Maritime Organization (IMO) goals. Nature may already have the optimized solution we’ve been struggling to build.
What Makes Cephalopod Pigments So Unique?
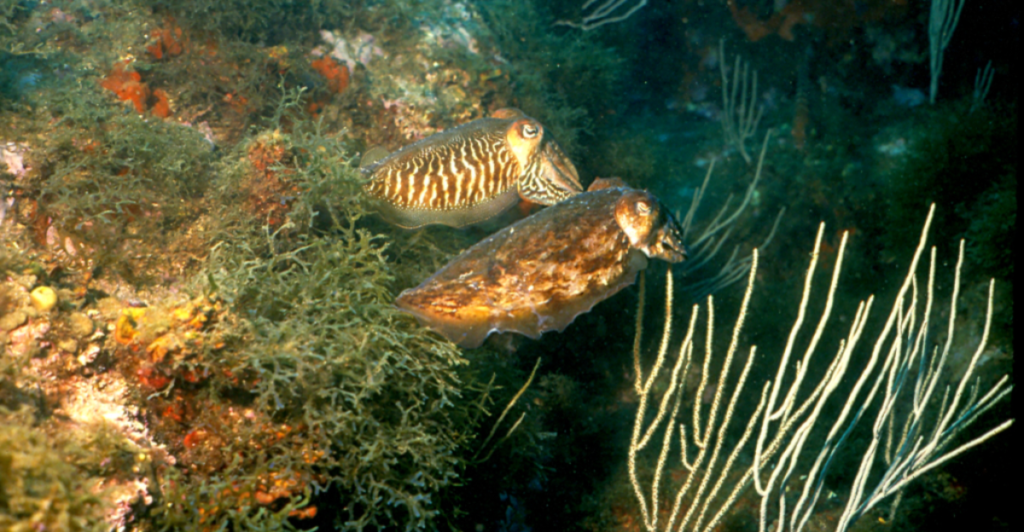
Unlike static pigments in most animals, cephalopods possess chromatophores that are dynamic, neuromuscular structures. These microscopic pigment sacs stretch and contract via precise muscle control, producing rapid, full-body color shifts.
Supplementing these are iridophores, which create shimmering hues through structural light interference, and leucophores that reflect surrounding colors. In hogfish, scientists like Heather Bracken-Grissom have found that photoreceptors underneath the skin provide real-time feedback, fine-tuning coloration with remarkable accuracy.
This feedback loop represents a biological photonic system that outperforms human-made sensors in speed and efficiency. It’s this same machinery that may be adapted to revolutionize solar power—transforming skin into a light-sensitive material that not only detects light but uses it to generate power.
Can Squid Pigments Really Generate Electricity?
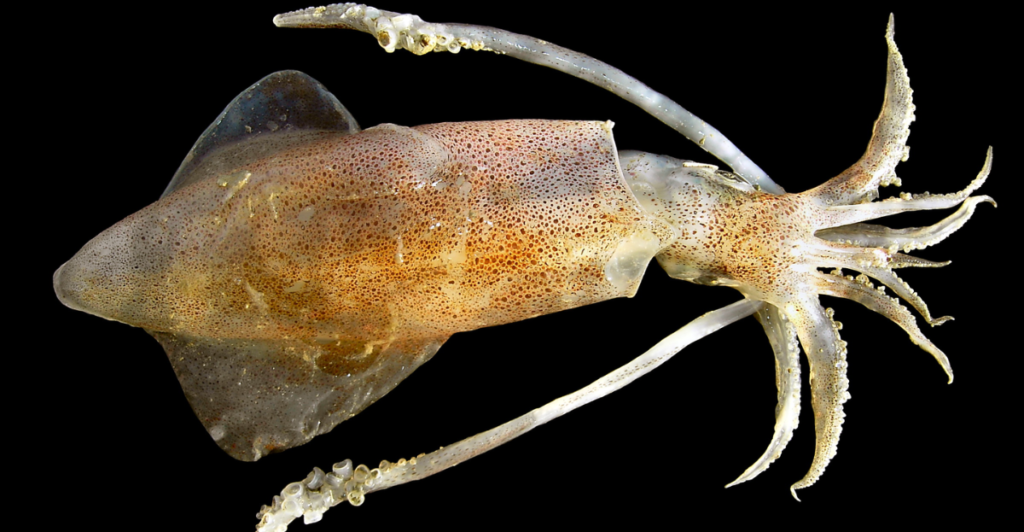
Yes—and with surprising promise. Leila Deravi’s team at Northeastern University discovered that squid pigment granules, when placed into photovoltaic cells, don’t just passively reflect light—they actively facilitate “photoexcited charge transfer.”
The more granules added, “the higher the photocurrent response is,” Deravi reports. Though 0.81±0.14% efficiency may seem modest, it’s groundbreaking considering the biological and water-based context. Unlike traditional solar materials, which falter under diffused or underwater light, these pigments thrive in low-light environments.
Cephalopods’ natural ability to shift color underwater hints at how efficiently they harvest light. Instead of reinventing the wheel, researchers are now asking: What if we borrowed the wheel nature’s been tuning for 500 million years? If scalable, these biomaterials could lead to flexible, adaptive solar tech ideal for marine vessels, submerged infrastructure, or even wearables.
Why Conventional Solar Panels Fall Short
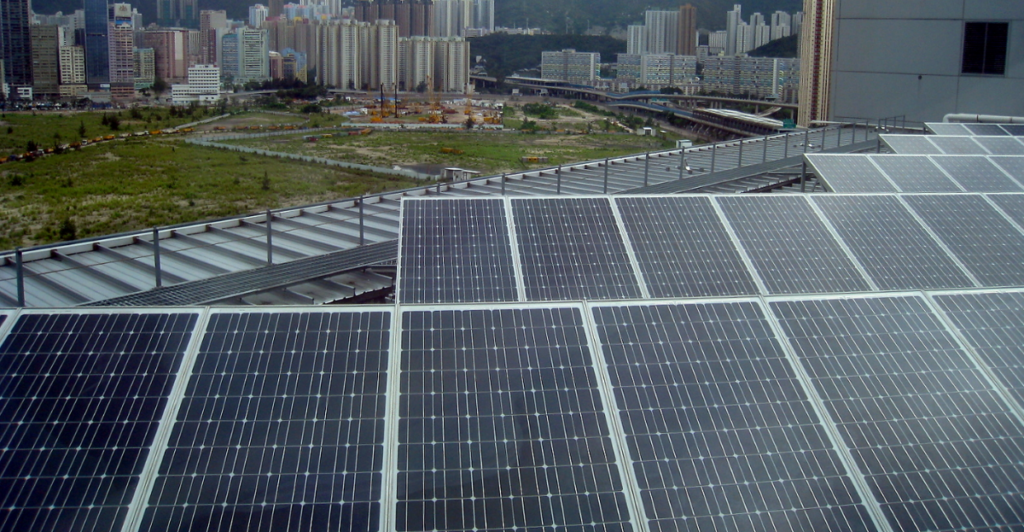
Today’s solar panels are hampered by a fundamental flaw—they’re inefficient in converting light to electricity. Commercial photovoltaic systems typically capture less than 25% of sunlight, with over 70% lost as heat. That waste not only reduces output but accelerates thermal degradation.
Worse, these rigid, white panels don’t integrate easily into non-rooftop environments. In maritime contexts, their utility diminishes further due to salt, motion, and variable lighting. Meanwhile, traditional desalination processes—crucial for ocean-bound sustainability—consume immense energy and dump brine waste.
With marine emissions making up nearly 3% of global CO₂, and energy-intensive operations pushing up fuel costs, the need for a rethink is clear. Engineers are now looking toward marine organisms, which evolved to optimize light use in underwater, fluctuating light fields—a vastly more complex task than what any solar panel faces above ground.
Are Giant Clams More Efficient Than Solar Panels?
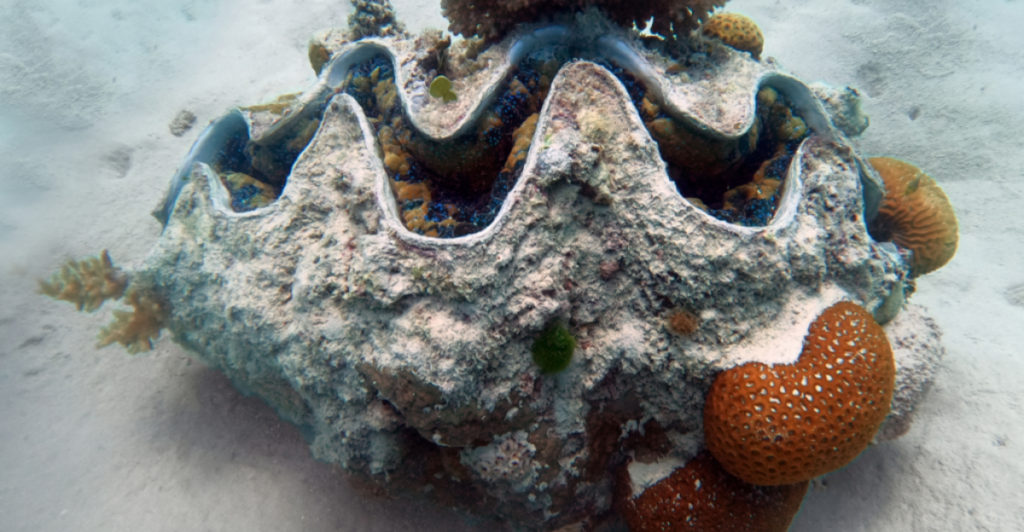
Surprisingly, yes. Giant clams use vertical columns of symbiotic algae and crystalline reflectors to optimize sunlight capture—achieving up to 67% quantum efficiency. That’s nearly three times more than average photovoltaic systems.
According to a Yale-led study, these creatures use light-scattering cells to illuminate algae evenly, allowing photosynthesis to occur without overheating. Other ocean dwellers like Synechococcus cyanobacteria use pigment-switching genes to adapt to changing underwater light, maximizing their energy production in varied conditions.
These natural tricks show us that efficiency isn’t about brute force—it’s about harmony with the environment. Marine life doesn’t just absorb light; it sculpts and sculpts it across time, shape, and color. These complex adaptations could form the basis of next-gen solar arrays that self-optimize across different settings—urban, aquatic, or atmospheric.
How Is Biomimicry Changing Solar Tech Design?
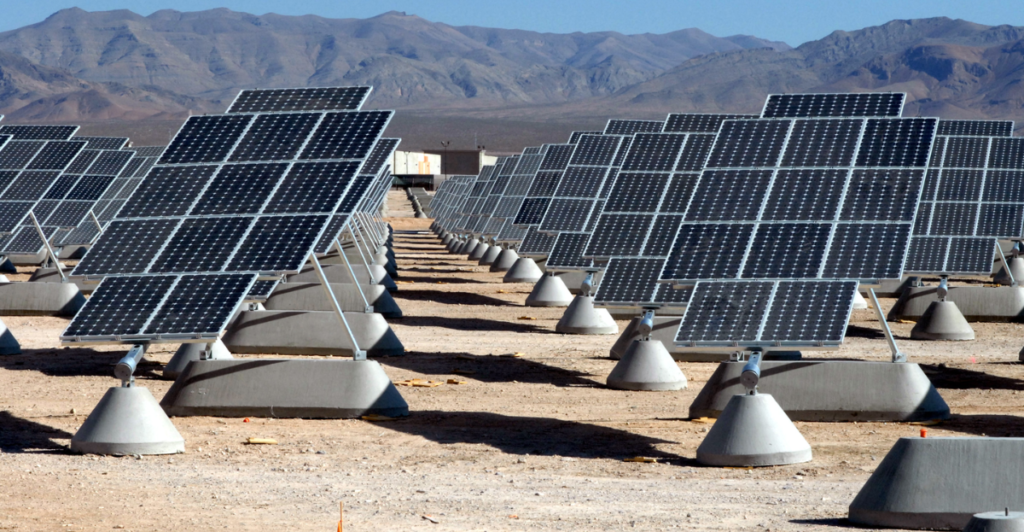
Biomimicry is transforming solar development by borrowing from evolutionary innovations. Scientists have combined plant light-harvesting systems with bacterial photosystems to create biohybrid devices that capture a broader spectrum of sunlight.
One promising invention is the “photovoltaic leaf,” which mimics plant transpiration to cool solar panels. By lowering temperature by ~26°C, it improves efficiency by 13.6%. Another marine-inspired breakthrough: mangrove-style solar distillers achieving 94% photothermal efficiency while producing drinkable water without brine discharge.
These examples prove nature isn’t just a source of inspiration—it’s a blueprint for scalable, sustainable design. Evolution has optimized energy collection under water, wind, heat, and shade. Our challenge now is not to out-engineer nature but to engineer alongside it, translating millennia of survival strategies into efficient, adaptable technology suited for real-world conditions.
What Makes Cephalopod Chromatophores Ideal Photosensitizers?
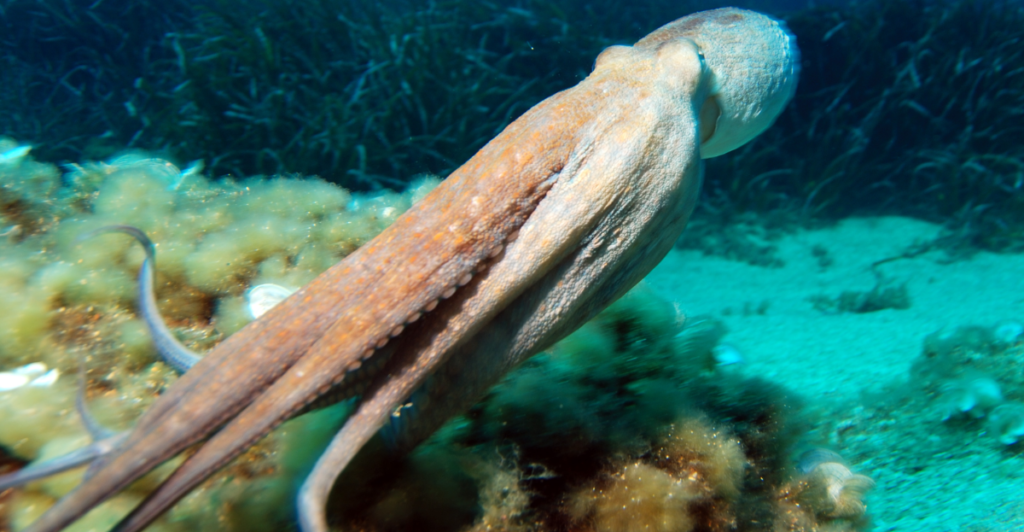
Cephalopod chromatophores are more than dynamic color pixels—they’re nanoscale light manipulators. Researchers found that chromatophore granules from the Doryteuthis pealeii squid function as “photosensitizers,” facilitating direct electron movement when illuminated.
What sets this apart from other pigments is its real-time adaptability: chromatophores adjust to surrounding light levels with exquisite control, even underwater. These same properties allow squids to communicate, camouflage, and now—potentially—generate power. Their nanostructures could be engineered into transparent or flexible photovoltaics capable of harvesting diffuse light, opening new possibilities for devices in shaded, submerged, or mobile conditions.
While the field is nascent, the paradigm shift is real. Biology is showing us that pigmentation, sensing, and energy conversion are not separate systems—they can be one elegant, multi-functional material.
Cross-Industry Impact: More Than Just Solar
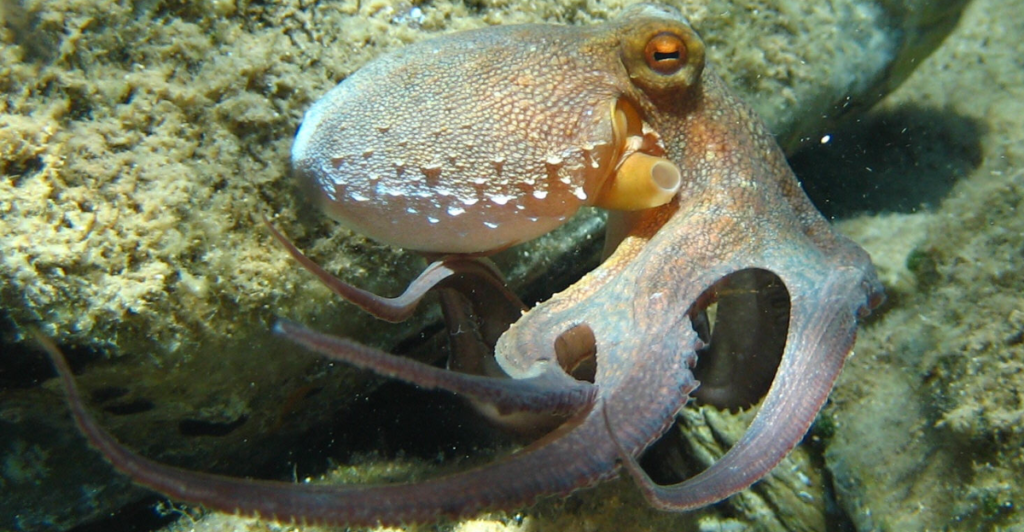
Cephalopod-inspired tech isn’t limited to energy. Harvard researchers are experimenting with squid-like building materials that regulate heat and light, potentially slashing the 25% of global energy use devoted to indoor climate control. In extreme climates, self-adaptive building exteriors could adjust reflectivity or color in real time—no electricity required.
Meanwhile, engineers have developed radiative cooling coatings inspired by thermochromic marine species, adjusting reflectivity based on temperature to reduce HVAC loads by up to 44%.
At the same time, marine-inspired water purification systems are also gaining traction, particularly those that use solar energy to produce fresh water and extract salt without generating brine waste. What started as a study of squid camouflage could fundamentally reshape the way we design homes, cities, and essential utilities.
What Are the Limits—and the Next Frontier?
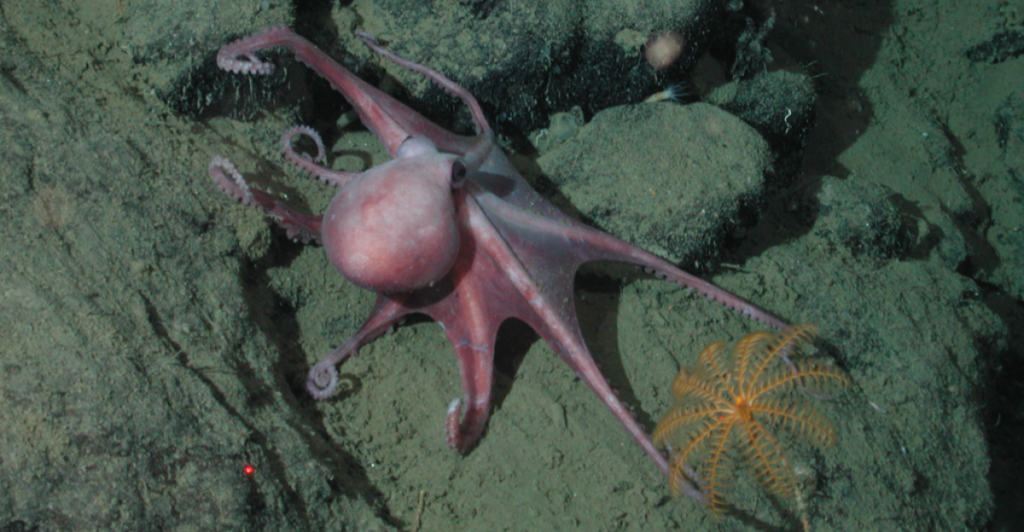
While promising, squid pigment solar cells are still in early stages. Their efficiency (~0.81%) is far below the 15–22% standard in commercial silicon panels. More research is needed to understand the precise mechanics behind underwater efficiency and whether these systems can be scaled or synthetically mimicked.
One mystery lies in the electrical signaling between chromatophores—currently observed at frequencies under 1.5 Hz—but the method of propagation remains unclear. Synthetic replication also faces material stability, cost, and environmental resilience issues.
However, combining squid pigments with conventional photovoltaics might yield hybrid systems tailored to challenging environments. For instance, vessels could use squid-based tech below deck or underwater, while standard panels operate topside. In smart cities, flexible, adaptive films could coat surfaces to harvest light dynamically, transforming how we think about solar integration.
The Deep-Sea Secret That Could Power Our Future
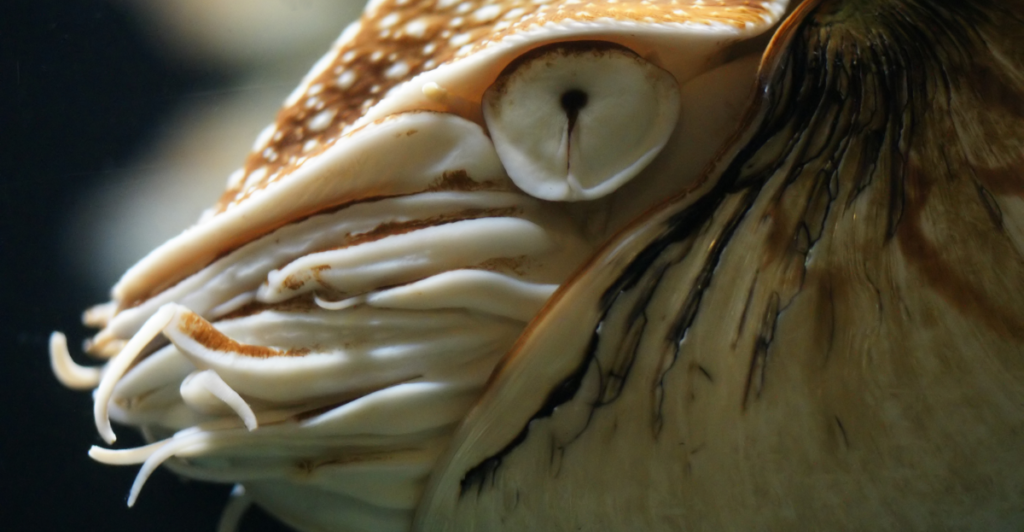
In a world racing to decarbonize, the ocean may offer an unexpected edge. Cephalopods, giant clams, and cyanobacteria have evolved light-manipulating systems far more refined than our best solar panels. As we look toward carbon neutrality, particularly in high-emission sectors like shipping and building, the pressure to innovate is mounting.
But innovation doesn’t always mean invention—it can also mean translation. The squid’s chromatophore might become our next solar building block, blending energy capture, thermal regulation, and sensing into one material.
Whether on smart windows, marine vessels, or wearable tech, color-shifting bio-solar hybrids could redefine the future of energy. Evolution has already solved many of our challenges. All we need to do is listen, learn, and build.
Explore more of our trending stories and hit Follow to keep them coming to your feed!
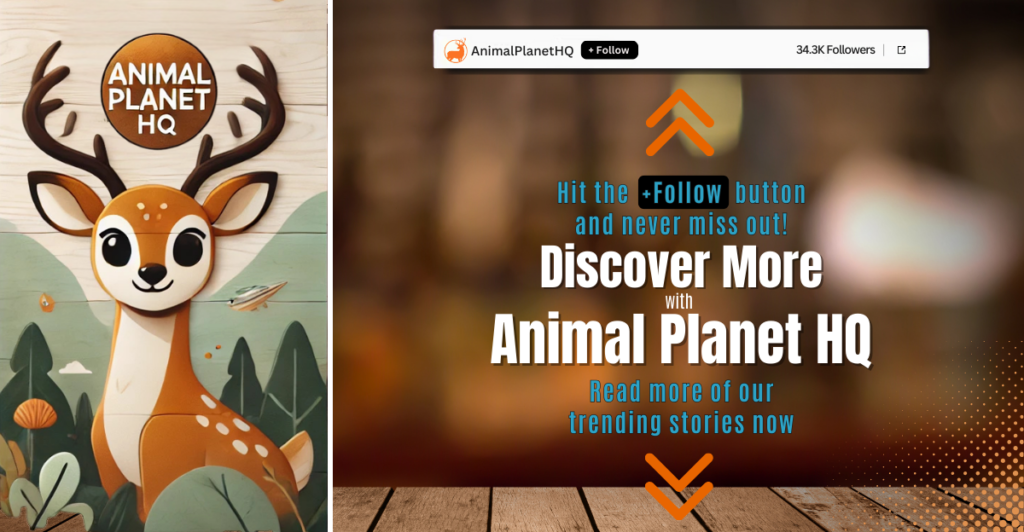
Don’t miss out on more stories like this! Hit the Follow button at the top of this article to stay updated with the latest news. Share your thoughts in the comments—we’d love to hear from you!